How Animal Hearing Works: Lessons from Owls, Mammals, and Auditory Systems
Discover how animal hearing systems work, from owls with their asymmetric ears to mammals' complex auditory processes. Learn how these natural mechanisms help detect sound and localize prey with precision, offering insights into biology and auditory science.
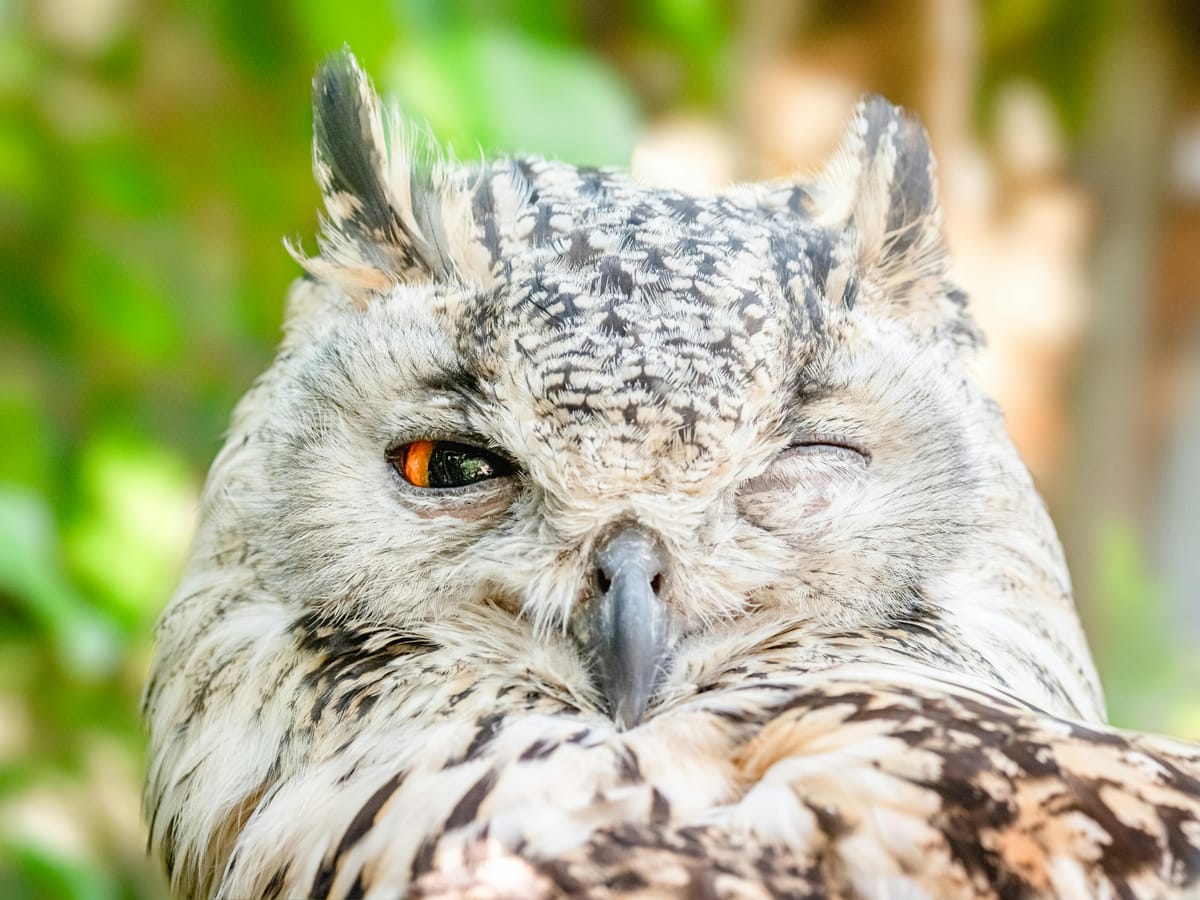
Hearing is one of the most vital senses in the animal kingdom. From hunting to communication, from navigation to avoiding predators, animals rely on a variety of auditory mechanisms to survive and thrive. In this article, we’ll explore the different structures and hearing abilities across various animal species.
Mammals, including humans, have a complex auditory system made up of the outer ear, middle ear, and inner ear. This system allows them to perceive a wide range of sound frequencies. We’ll get back to this shortly.
Birds, on the other hand, have sharp hearing that helps them in communication and sound localization. Owls, for instance, have asymmetrical ears that allow them to precisely locate prey both vertically and horizontally. I’ll revisit this fascinating topic soon!
Amphibians and reptiles have less developed, but still effective, auditory structures. A fun fact for puzzle enthusiasts: snakes, which lack external ears, perceive ground vibrations through their jaw bones. And another fun fact for those who, like me, wonder if fish drink or sweat: some species have a lateral line, a sensory organ that detects vibrations in water.
The mammalian auditory system is a highly sophisticated set of mechanisms designed to convert sound waves into electrical signals that the brain can interpret. It is composed of three key elements: the pinna (outer ear), the middle ear, and the inner ear. These elements work together to process sound input effectively and accurately.
The pinna, the visible part of the ear, acts like a natural antenna, collecting sound waves from the environment and channeling them through the ear canal. Its shape is far from random; it is designed to concentrate and direct sounds inward. Even at this stage, a preliminary filtering of sounds occurs, based on direction and intensity—like a natural filter.
Once sound waves enter the ear canal, they hit the eardrum, a thin and flexible membrane that acts as a transducer, converting sound waves into mechanical vibrations. This is a crucial step: vibrations are the format that can be processed and transmitted further. The vibrations of the eardrum are then transferred to the ossicles—malleus, incus, and stapes—a lever system that amplifies the signal and directs it to the inner ear. Despite being the smallest bones in the human body, the ossicles play a crucial role in increasing the signal’s intensity, keeping the sound strong and clear. In addition to this, they protect the inner ear thanks to a reflex that, in the presence of very loud sounds, limits the movement of the bones to prevent damage to the delicate inner structures.
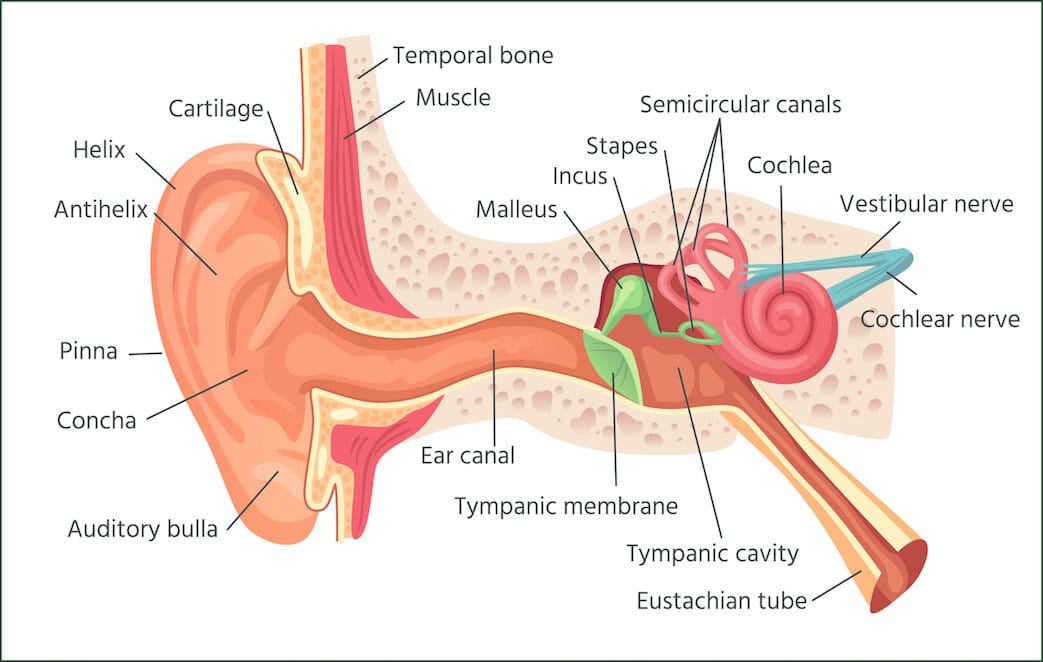
The Eustachian tube, also located in the middle ear, is another important component. This canal connects the middle ear to the throat, maintaining the balance of air pressure between the inside and outside of the ear. Proper balance is essential for the eardrum to function correctly. If the pressure is imbalanced, as it often happens when your ears “pop” on a plane, the eardrum can’t vibrate effectively, compromising the entire sound transmission process.
The inner ear is the most critical part of the system—a true "input system" that converts vibrations into electrical signals that the brain can interpret. The central element is the cochlea, a spiral-shaped structure filled with fluid. The vibrations generated in the middle ear cause the fluid to move within the cochlea, activating a membrane called the basilar membrane. This membrane vibrates in response to different sound frequencies: low frequencies make the apical part vibrate, while high frequencies affect the basal part. In this way, the cochlea acts as a filter, separating the different frequencies and providing an initial "cleaning" of the signal.
On the basilar membrane are hair cells, equipped with tiny projections called stereocilia. These sensory cells are the true transducers of the signal: when the stereocilia move due to vibrations, ion channels open, generating electrical impulses. These electrical signals travel through the auditory nerve to the brain, where they are interpreted as sounds.
One interesting aspect, which relates to my work in cybersecurity, is how the auditory system adopts measures to validate and filter input. Hair cells don’t work in isolation; they receive feedback through the olivocochlear efferent system, which sends signals from the brain back to the cochlea to regulate the sensitivity of the cells. This feedback mechanism helps filter out background noise and irrelevant sounds, much like a system that checks the integrity of input, discarding invalid signals. The auditory system doesn’t passively collect sounds; it actively controls the sensitivity of the hair cells depending on the context.
While writing this article, I realized how similar the auditory system is to input management in cybersecurity. Sound input, like any data, must be handled carefully, ensuring the Confidentiality, Integrity, and Availability of the information. I can’t help but draw parallels between these two worlds.
Filtering and selective amplification directly affect Availability (the ability to access relevant sounds both now and in the future) and Integrity (filtering out unwanted frequencies improves the clarity of the desired signal). For example, the pinna and ear canal act as natural filters that dampen unwanted noise, while the ossicles selectively amplify vibrations, improving sensitivity to important sounds and reducing background noise.
Another essential protection concerns protection from harmful input: the acoustic reflex, which kicks in when the sound is too loud, reducing the movement of the ossicles and protecting the inner ear, much like a firewall blocking harmful traffic.
Validation and advanced processing are carried out by the hair cells, each of which is sensitive to a specific frequency range. This ensures that only valid input is transmitted to the brain. If you know me, you’ll know how much I insist on input validation: the auditory system is no exception, implementing dynamic adaptation that adjusts sensitivity based on context. Finally, the neural feedback system sends signals from the inner ear to the brain to adjust perception in real time, similar to a firewall updating its rules to filter traffic optimally.
And to conclude, there’s always the "What are you even saying?"—an ambiguous system of repetition that could be compared to a security system that does everything and nothing, like the CISO in almost every company I’ve seen over the years.
As promised, let’s talk about the adorable panoramic Strigidae (aka owls! Panoramic because they can rotate their heads up to 270 degrees).
Owls have a unique adaptation in their auditory system: their ear openings (the internal ears) are asymmetrically positioned on their skull. This means one ear is slightly higher or lower than the other, or positioned further forward or backward. It’s important to note that this asymmetry doesn’t involve the "ear tufts" many people think are ears (they are just decorative feathers!), but the actual ear openings.
What happens on the horizontal axis (Left-Right)? When a sound comes from one side, it reaches the closest ear slightly earlier and with greater intensity than the other. The owl’s brain uses this difference in timing and intensity to precisely determine the horizontal direction of the sound source.
Regarding the vertical axis (Up-Down), thanks to the vertical asymmetry of the ears (one higher than the other), the owl can detect sounds coming from above or below. The higher ear will pick up sounds from above with greater intensity and slightly earlier than the lower ear, and vice versa. This allows the owl to pinpoint the vertical position of its prey.
In practice, imagine a mouse moving through tall grass. The noise made by the mouse generates sound waves that reach the owl’s ears. Due to the asymmetry of the ears, there’s a slight difference in the time of arrival and intensity of the sound between the two ears. The owl’s brain processes these differences, accurately calculating the exact position of the mouse both horizontally and vertically. The owl can then dive in with millimetre precision and catch its prey without missing a beat.
And this wraps up the preliminary discussion on how we 'hear' sounds. In the next post, we will answer the question, 'Why do we only have seven notes?"